Radiopharma: Basic Principles, Opportunities
Hot take: Radiopharm > ADCs. Relevant for LNTH, NVS, PNT(now Lilly), FUSN, ATNM, RYZE
Hello everyone! Before diving into the article, I would like to introduce a new project of mine, www.subradata.com. The website is designed to keep investors updated on changes to clinical trials, new medical guidelines, and any website changes. Additionally, two work-in-progress features will allow users to A) track management commentary across multiple press releases and b) view recently updated drug labels.
"There are three ways to make a living in this business: be first; be smarter; or cheat. Now, I don't cheat. And although I like to think we have some pretty smart people in this building, it sure is a hell of a lot easier to just be first." - Margin Call.
I would recommend signing up if you’re curious. No spam, no ads, and free to sign up. It’s also your choice to sign up for email notifications, but if you do you can look forward to one email per day on clinical trial updates for your companies and/or an email whenever new guidelines are posted, and/or a website changes. You can even switch notifications off at any time. Again, it’s all based on your choice and if you have any feedback, just email me.
Maybe you'll see something that gives you an edge.
Investing shouldn't be a measure of resources; it should be a measure of wit and the website is meant to level the playing field between large funds with access to sophisticated tools and equally intelligent individuals pressed for time competing against those funds. The site is messy, not user friendly, but functional. Play around. See what you can and can’t do and please contact me with questions and feedback.
If you have any questions, comments, or feedback, please contact me at adu@subradata.com or adu.subramanian@gmail.com.
The Post Begins here.
Introduction:
I was planning on doing a series where I review PNT, ATNM, FUSN, RYZE, NVS, and LNTH, but the BD folks at LLY and PNT seemed to have other plans. Nevertheless, I find radioligand therapy (RLT, also known as radiopharmaceutical therapy) an intriguing, dynamic, emerging modality for many reasons. The commercial history of most drugs has been disappointing, but Novartis has shown how one therapy can scale. Perverse incentives and marginally beneficial results over standard of care obscured the potential for radiopharmaceuticals, and I believe we're turning a corner. In this series, I'll cover the history, the current generation, and the future of radiopharma. I'll also discuss the basic physics, supply chain, and scientific considerations, both in terms of physics and biology. Let's dive in.
Basics:
Radiopharmaceutical therapy works by administering a radioactive compound to the patient. The compound is designed to target specific cells or areas in the body, such as cancer cells or overactive thyroid tissue. Once inside the targeted region, the radioactive material emits radiation that damages or kills the unhealthy cells while sparing nearby healthy tissue. This targeted radiation helps treat the disease, like cancer, by reducing or eliminating the abnormal cells. Medical professionals carefully control the dose to ensure effectiveness and minimize side effects.
History
The first two approved radiopharmaceutical products were Bexxar (Iodine-131 CD20 RPT) and Zevalin (Yttrium-90 CD20 RPT), both of which failed commercially for numerous reasons (Bexxar was actually taken off the market in 2014). Both were initially approved for refractory Non-Hodgkin's lymphoma post rituximab, and Zevalin was approved for first-line consolidation therapy for patients who had some response to NHL chemo but no rituximab. The standard of care was rituximab, and neither therapy differentiated itself in terms of efficacy/safety, so the patient population was restricted. Furthermore, there were economic reasons for their commercial failure. An article from 2007 details lackluster reimbursement where Medicare reimbursed hospitals $16k for a $25k treatment (link). In addition, oncologists had to refer patients to radiation oncologists and lose lucrative revenue through rituximab infusions. Patient-specific dosimetry (the need to measure the dose in each patient), minimal shelf-life of Iodine-131, instability of the companies, and a lack of capability outside of specific centers further hampered sales (link). A 2010 survey of physicians sums it up nicely: "Radioimmunotherapy was generally viewed positively by the surveyed population. However, limited referrals due to alternative nonradioactive therapies and logistic, educational, and economic concerns played an important role for subgroups in the perception of radioimmunotherapy for NHL." (link)
The next generation: Xofigo, Lutathera, Azedra.
These therapies target solid tumors and have had some success so far. I'll focus on Xofigo and Lutathera because Azedra was approved for a niche indication and recently discontinued production.
Xofigo (Radium-223 di-chloride): It’s an alpha-emitting treatment for prostate cancer patients with ONLY bone metastases. Xofigo isn't truly targeted because radium mimics calcium and is preferentially taken up by bone cells, not targeted using a ligand. It's dosed at 50 kBq/kg every 4 weeks for 6 injections (total dose ~21 MBq per 70 Kg person) (link). Xofigo has been moderately successful in a small population (patients with only bone metastases and no soft tissue progression). Xofigo, unlike Bexxar and Zevalin, can be administered by oncologists (though most is still done by radiation oncologists) and generates $250 million in sales per year. The same logistical challenges exist, and efficacy is still marginal. Most patients aren't eligible for therapy and will receive other options such as androgen deprivation therapy, linear accelerator-based radiation, or chemotherapy.
Lutathera (LU-177 Dotatate): a Somatostatin receptor analog (SSTR) developed by Advanced Accelerator Application and acquired by Novartis in Jan 2018 post-approval for the treatment of GEP-Neuroendocrine tumors (NETs).
Dosage: It's dosed at 7.4 GBq Q8W for 4 doses and followed by a long acting octreotide administered <1 day after each dose. The label + dosimetry can be found here.
Benefit: Median PFS, ORR, and OS all demolished the standard Octreotide group (see Table 8), thus the therapy has been commercially successful with ~500M in FY2023 sales and peak projections up to 750M in 2027. Novartis recently announced a success trial comparing to 1L SOC therapy (link).
Pluvicto: The big dog. It’s a PSMA targeted Lu-177 therapy which proved the commercial viability (and potential issues) of radiopharmaceutical therapy.
Dosing and Benefit: it’s dosed for 6 cycles of 7.4 GBq every 6 weeks (label) and currently approved for prostate cancer patients after chemotherapy ('post taxane'). Novartis will present results in the pre chemo population soon and we know met the primary PFS endpoint, but approval was delayed because the FDA requested more details on OS.
Supply chain issues: Pluvicto sales blew analyst estimates out of the water (nearly a 1B drug in the first year) but supply chain constraints prevented every patient from receiving therapy. Radiopharmaceutical manufacturing requires expertise, infrastructure, and logistical coordination to run smoothly. Novartis is actively taking steps to open new production, but severe shortages prevented using the drug for weeks.
Radiopharmaceuticals: First Principles and Future Prospects
Radiopharmaceuticals are a powerful class of therapies that utilize radiation to target and treat various diseases, particularly cancer. These therapies are built on several key principles that distinguish them from other treatment modalities.
Molecules, Radiation, effects:
Three types of radiation are commonly employed in radiopharmaceuticals: alpha, beta, and gamma. Gamma radiation has the highest energy, but alpha and beta particles are preferred in Radiopharmaceutical Targeted Therapy (RLT) because they do not readily pass through the human body. It's essential that a therapy releases all its energy within the body.
A few key characteristics:
Beta radiation has a lower Linear Energy Transfer (LET), meaning it loses energy gradually over distance, making it akin to a bullet. In contrast, alpha radiation is more like a wrecking ball due to its larger size, consisting of two protons and two neutrons. This results in alpha radiation losing energy rapidly over a shorter distance. Consequently, alpha radiation is better suited for micro metastatic diseases, while beta radiation may be more effective for large tumors. (source)
Alpha particles also decay to multiple alpha emitting particles while beta decay decays to a stable nucleotide so we must consider the decay chain of alpha particles to ensure patient safety.
Alpha particles kill cells via clustered double strand breaks while beta cells create single strand DNA breaks with less lethality (source). Returning to the wrecking ball analogy, the wrecking ball is more damaging than a bullet.
Alpha his a higher Relative biological effectiveness: the ratio between dose of reference radiation to dose examined. It give you more bang per dose administered.
Therapy construction:
A radiopharmaceutical comprises three essential components: a targeting moiety, a chelator/linker, and a radioactive element.
Chelator/linker: the simplest, yet hardest to engineer, piece is the chelator which attaches the element to the targeting vector. The industry standard chelator is DOTA based (stands for 1,4,7,10-tetraazacyclododecane-1,4,7,10-tetraacetic acid) and both Pluvicto and Lutathera use a version of DOTA. The chelator + linker serve two purposes:
Bind tightly to the radioactive nuclide so it's not released. Bad stuff happens with untargeted therapy!
Modify the pharmacokinetics. We want the pharmacokinetics to match the half-life of the isotope so the linker is key for effective drug development.
Radioactive elements: The most common nuclides in development are Actinium-225(T1/2 ~10 days, alpha emitter) and Lutetium-177 (T1/2 = 7 days, beta emitter). Each are chosen for their ideal half-life (between 5-10 days), experience with production, and minimal gamma radiation (less shielding required). Isotope choice depends on A) half-life of emission B) well-studied chemistry and C) ease of production. Radiopharmaceutical chemistry and isotope selection are areas of ongoing development, even with commercial therapies available.
Targeting vector: An ideal radiopharmaceutical targets vectors that remain in the tumor for an extended period, penetrate deeply, and are excreted quickly from the body without accumulating in the kidneys. We want a target solely expressed in tumor, but do not require internalization (unlike ADCs). Monoclonal Antibodies, while easy to manufacture for high affinity, have limitations due to their large size and long retention time In the body (in part due to FcRn recycling) (source). Alternative vectors include small peptides, small molecules (like Pluvicto), and antibody fragments. Small molecules are on the other end of the spectrum with short half-lives and fast excretion. Both antibodies and small molecules can work, but the shorter retention time of small molecules is ideal for nuclides with short half-lives and vice versa.
(source if you want a comprehensive overview)
Drug Development and Dosimetry:
Dosimetry, or the measurement of absorbed radiation dose in both the tumor and healthy tissues, is a crucial aspect of radiopharmaceutical development. The goal is to deliver an effective dose to the tumor while minimizing damage to surrounding tissues. Administered dose is measured in Becquerels, while absorbed dose is measured in Grays. Dosimetry is key to guiding dose escalation during clinical trials.
Radiopharmaceuticals are guided by toxicity where trials dose escalate based on off target absorbed dose.
Current limits to healthy organs:
Dose Response: More dose = Better response.
Evidence from radiation oncology and clinical results supports a dose-response relationship between the absorbed dose in the tumor and tumor response. For example, PSMA-Lu Therapy shows a better response when the absorbed dose to the tumor exceeds 10 Gray. (source)
Lutathera shows a somewhat asymptotic limit for absorbed dose to the tumor where any absorbed dose above 100-200Gy had only marginally better results.
We can see a clear correlation and leveling off of absorbed dose to the tumor and the best response in tumors of size >2cm (A) and size >4cm (B). The relationship is still unclear and evidence is building. However, the principle of "punch the tumor, don't hit anything else".
Dosimetry: Alpha vs Beta:
I'll note the published dosimetry data is primarily for beta emitters and the low LET for alpha emitters makes dosimetry much harder for alpha therapies (among other reasons). We have to account for each element in the decay chain and perform dosimetry on a much smaller scale. When the radiation only reaches a few cells, measuring dose delivered is more difficult and presents new challenges. I still believe whole tumor absorbed dose is a good metric as a proxy for efficacy.
Abscopal effect:
RLT, in addition to direct DNA damage induces an "abscopal effect" to activate the immune system and kill neighboring cells. Alpha therapies in large tumors hinges on such a phenomena because the radiation doesn’t penetration the whole tumor. Trials are underway with no positive results yet. All published research is from external beam radiation.
Supply chain:
The supply chain for radiopharmaceuticals, particularly Lutetium-177 and Actinium-225, is a critical factor in their successful clinical deployment. Supply chain challenges can significantly hinder commercial rollout so companies like Novartis and Point are expanding production capacity to meet growing demand.
Lutetium-177:
Two ways to make Lu-177:
Direct neutron capture from Lu-176, aka carrier added Lutetium. Lutathera used this approach because it’s a high probability reaction (only 1mg Lu-176 for 50 doses lutathera) and processing is simple. However, only 30% is useful Lu177 at the end and some Lu177m is produced which requires extensive waste management in physician offices (the latter hinders commercial uptake)
Irradiation of Yb-176: This approach alleviates the waste management and low yield issues, but requires much more Yb-176 per patient dose and is harder to separate. (source). Novartis has transitioned Lutathera to this method of production. This route requires a reliable Yb-176 supplier and expertise in production.
Plants opening to meet demand: both Novartis and Point are opening plants to meet Lutetium demand. Novartis is targeting a 250k dose capacity by 2024 (equal to 40k patients or 10B in revenue)
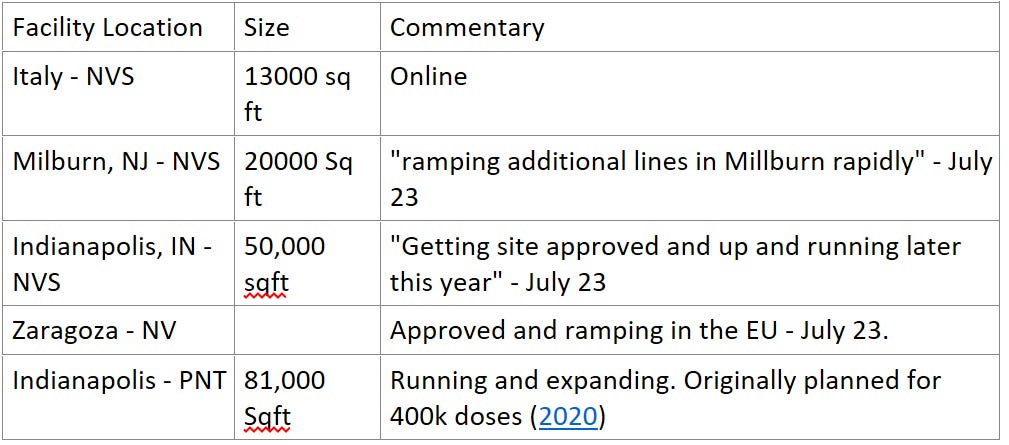
Producing Lutetium is difficult and requires domain expertise. This isn't a small molecule drug plant. Each dose has a limited therapeutic window for shipment so logistics are equally important as clinical data and science. Both Novartis and Point have a unique manufacturing expertise which can't be replicated (why LLY likely bought PNT, kudos to @adar17 on Twitter)
Actinium-225:
Oh lord, we do NOT have enough space to review the whole supply chain effort for Actinium production so I'll provide an overview and my bottom line thoughts.
One patient dose of Ac-225 is ~.5 millicurie and the current supply is about 2 Ci/per year. If we want to treat 10,000 patients, we will likely need 10 Curie (a 5x from current levels), nowhere near our current supply. If Actinium therapies become SOC, we will need hundreds of curies. A crisis could be imminent so the DOE and private sector has been working to proactively to increase supply (source). The sole supplier is currently the DOE via Thorium generators, but a few companies are working to diversify production.
Production starts with either Thorium 229 (via U-233, built up during the Manhattan project) or via Radium-226. (see Table 1). Th-229 production is the only method to currently make Ac-225, but Ra-226 production is key for the future. National labs focus on Th-229 and private companies are using electron beam radiation of Ra-226 to generate Ac-225 (among other methods). Numerous methods are in development, but no company is beyond proof of concept. One key limiting factor is the supply of Ra-226, the OG radioactive isotope (killed Marie curie!). Governments realized putting radiation in our watches isn't great so they phased out use of Ra-226. Current sources include some older medical/industrial facilities and uranium ore mining. The current supply of Ra-226 should be enough for Ac-225 therapy, but it could be a rat-race to secure reliable sources if Ac-225 becomes clinically successful.
Lead-212:
Lead-212 is an emerging radionuclide with unique properties, including a short half-life that requires decentralized manufacturing but matches small molecule pharmacokinetics. Companies like ArtBio are developing production platforms for Lead-212. It can also be imaged directly thus it can be a true 'theranostic' (therapy and diagnostic in one). Manufacturing is still early stage, but I eagerly await future developments.
The bottom line: Radiopharmaceutical production requires specialized expertise due to the limited shelf life of these isotopes. Supply chain reliability will be a critical differentiator in the future as we ramp up production of novel therapies.
Why I believe Radiopharmaceuticals are an advantageous modality:
Advantage 1: Dosimetry turns biology into physics. IO is a minefield of early stage results not translating in larger trials because it's a biology problem. Dosimetry is a physics problem: we need to deliver a dose to the tumor to kill it. Can we get that dose without off target toxicity? Early stage, in-human dosimetry translate well into late stage results for efficacy (with small N ~10-20). Comparable patient populations should show comparable dosimetry results and dose = response.
Advantage 2: The unique manufacturing requirements of radiopharmaceuticals make it challenging for generic entry. Companies that develop effective therapies can expect long-lasting revenue streams. One potential downside is IRA price negotiations on small molecules, but I believe therapies showing a strong clinical benefit will be reimbursed appropriately.
Advantage 3 vs ADCs: Radiopharmaceuticals sound like a more logistically complicated ADC, but they're not the same. ADCs require internalization, cleavage and cells have escape mechanisms to the payload itself (link). Radiopharmaceuticals offer a more straightforward treatment approach compared to Antibody-Drug Conjugates (ADCs). They do not require internalization or face the complex challenges of cellular drug delivery mechanisms.
Where is the future going?
The future of radiopharmaceuticals likely involves combination therapy with other modalities, such as immunotherapy, DNA damage therapies (Ideal for Lu-177 because it works via DNA damage), and possibly ADCs. Alpha therapies will likely move from second-line treatment to first-line, but Lutetium-177 therapy will still play a crucial role due to its scalability and effectiveness against large tumors. Developing new chelators, linkers, and ensuring a robust supply chain will be key sources of competitive advantage.
Companies I like:
PNT (LLY now) - Strong manufacturing, SPLASH should be positive, FAP program given minimal credit
LNTH - SPLASH readout upcoming, Potential reimbursement improvement, new tau based antibodies for imaging
NVS - building capacity for increasing Pluvicto usage. Leader in the space.
Neutral: FUSN - Supply chain is primarily DOE and results are too early even though they are the farthest along.
Dislike: ATNM - Iodine administration is difficult and OS results aren't strong enough to justify Iomab Use.
RYZE - long phase 3 trials with unclear targets and production.
cellectar bioscience CLRB has phospolipid drug conjugate which attack cancer cells through the lipid raft of the cancer cells , the PDC can carry any kind of payload from small molecule , peptide , radio isotope etc. because the lipid raft is present in all kind of cancer cells that mean CLRB drugs can target every kind of cancer, wonder why the author doesn't mention CLRB